History of British radar tubes
History of British radar tubes
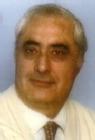
Foreword: The development of electronic sets and of related vacuum tubes was greatly accelerated in the years just before the Second World War and during the war itself. The most obvious progress regarded radio-localization (radar) systems. In the mid-thirties the upper limit of the radio frequency was slightly higher than 30 MHz while only ten years later, in 1945, radar systems operating at 24 GHz were currently in use. A comparison between the countless systems developed over about ten years by belligerent countries is in fact impossible: yesterday details were strictly secreted, today old systems have been scrapped and in any case it would be impossible to put them back into operation. Nevertheless we have today a reliable way to reconstruct radar history, simply looking at the evolution of radar-specific vacuum tubes designed over the years, mainly for the transmitter. Details of vacuum tubes unveil essential performance of systems in which they were used.
This serial overview is mainly focused on British tubes simply because England set most of the standards for modern radar systems, constantly proposing and evaluating new solutions and new components. America played a decisive role in the industrialization and volume production of radar sets, related components and ancillary equipment, but their origin was in England. In that country were devised many VHF / UHF sealed-disc or external anode tubes, the cavity magnetron, the reflex klystron, the magnetron strapping, the silicon mixer diode, up to the T/R switch and the memory CRT. Even the traveling wave tube, although this only appeared after the end of the war, was devised at Clarendon. Germany probably had a small advantage on UHF triodes at the start of the war but failed to invest in subsequent developments in higher frequency and microwave devices.
Trying to investigate the history of radiolocation systems in the 1930s, too often are overestimated episodic facts related to simple filing of patents or to experiments intended solely to prove the feasibility of radio-detection. The system that was emerging had to give information about the position of passive obstacles or targets. It could be quite similar to a radio-compass, widely used since the early twenties, with the addition of a self-contained RF transmitter to illuminate targets. The reflection of radio waves by obstacles was well known at the time and this property could be used to 'see' where sight could not reach. Experiments were oriented to systems capable to avoid collisions or allow landings in the fog, or even to see enemy ships or aircraft and direct artillery fire. Since the radio signal had to travel twice the distance of the target, from the transmitter to the target and back to the receiver, its attenuation was proportional to the fourth power of the distance itself. Use of high-frequency beams was preferable since it was easier to build lightweight directional antennas at short wavelengths. Then new transmitting electron tubes were required, capable of generating strong RF pulses at the highest frequencies. These two conditions were antithetical in the 1930s, when most power tubes could not operate above a few megahertz. Several feasibility studies on UHF radar systems were conducted almost simultaneously in many countries since the mid-thirties of the last century, using still experimental components. The state of the art for high-frequency transmitting tubes in those years is given by several publications, as the article by Kelly and Samuel appeared in the November 1934 issue of Electrical Engineering (Bell System Technical Journal 14.1.97) or the technical articles given in the RCA book ‘Radio at Ultra-High Frequencies’.
The upper frequency limit of modern transmitting triodes was somewhere around 100 MHz. The common design philosophy to minimize parasitic parameters was to keep tube dimensions small. This way led to severe limits, both in the maximum working voltage and in power dissipation, even in transmitting tubes designed for frequencies just above 100 megahertz, which did not exceed one hundred watts. Higher frequencies could be obtained from triodes operated with positive grid, as proposed by Barkhausen-Kurz. Unfortunately their efficiency was low, around 5%. Furthermore grids were not designed to dissipate heat resulting from impact of electrons. Lindenbland in 1935 wrote of a 6 watt transmitter at 462 MHz, using two UX-852 power triodes in push-pull. Two 846 water-cooled triodes - over 1.1 kilowatt wasted only for heaters - were used to generate 115 watt at 411 MHz. The most efficient source in UHF region was the magnetron. Anyway its usefulness as high power source was very poor, due to the low emission of the filamentary tungsten cathodes and even to the poor heath dissipation from the plate segments, usually surrounded at the time by the evacuated glass bulb. In the mid thirties the best performing types were of the split-anode type, operating somewhere between 500 and 700 MHz and rated for CW output power of about 50 watt.
Fig. 1 - Some of the tubes available around the mid 1930s for high frequency operation. A) The ‘acorn’ 955, introduced by RCA in 1934, was very small, designed to operate as signal amplifier or low-power oscillator up to 600 MHz. B) The Western Electric 384A pentode was another example of small high-frequency receiving tubes, the mushroom family. The family included octal based types, as the 717A. Shortly later the family was rebased to 7-pin, originating universally used types, as the 6AK5. C) The 852, introduced in 1927, used a short and large plate to keep interelectrode capacitances low. D) WE 304A was designed in 1934 to operate up to 350 MHz. The sample in the photo is the Philips equivalent TB1/60, with graphite anode. E) The water-cooled triode 846 was introduced in 1932. Actually it was specified for 50 MHz full ratings but was sometimes used as Barkhausen-Kurz oscillator in the UHF region. F) The MOV CW10 magnetron, designed by E.C.S. Megaw, was advertised from 1936. Its output power was somewhere between 10 and 30 watt, depending upon the wavelength. A similar device was used by Gutton in France, who continued experimenting interdigital magnetrons in the low-power anti-collision system first installed on board of the Normandie liner. The system was just intended to sense obstacles straight ahead of the bow, so requiring little radiated power.
In November 1934 a paper published in Electrical Engineering and reprinted on the Bell System Technical Journal compared the decreasing size, and consequently the decreasing plate power, of transmitting tubes designed to operate at increasing frequencies.
Fig. 2 - A) Transmitting tubes for increasing frequencies at subsequent decreasing power. From left, 1 kW air-cooled transmitting tube for short waves, the industry standard 852 usable up to 180 MHz, the 304A usable up to 350 MHz. The last two triodes were experimental samples, the last one usable above 1 GHz and delivering 1 watt RF. In B) close-up view of one UHF experimental prototype. Source Bell Telephone Technical Journal.
The UHF triode visible in fig. 2B would have a considerable impact on early experiments with UHF radio-localization in many countries. We will see that in Germany it led to the development of variants produced in volume for operational radar sets. In August 1936 Western Electric introduced the 316A, the first doorknob-shaped UHF power triode based upon that electrode structure. At the time it was capable of delivering 30 W at 500 MHz and of oscillating up to 750 MHz.
Fig. 3 - Some doorknob UHF power triodes from Western Electric. A) The 316A was first introduced in 1936. It was derived from the experimental type of fig. 2B and could be used up to 750 MHz. B) The double-ended 368A was intended to be mounted in the middle of the resonating lines, oscillating up to 1.750 MHz. C) The 388A was a larger and more powerful double-ended triode with graphite anode. It was capable of oscillating up to 900 MHz, with 50 W plate dissipation.
In America Western Electric used doorknob tubes until the early 1941 in the CXAS experimental radar. Two triodes generated the marginal peak power of 2 kW at about 700 MHz. The transmitter was then uprated using a cavity magnetron and subsequently doorknobs were confined to applications requiring quite low power, as IFF transponders and radar jammers.
In England Bowen tells of his experiments with WE 316A in airborne radars. He started in March 1937 with a single tube oscillator. Few months later his group designed a transmitter with a push-pull of 316As, delivering 1 kW pulses at 240 MHz. Test flights evidenced that the ASV system was less affected by sea-clutter at lower frequencies, around 200 MHz. As consequence, the transmitter was modified to use a push-pull of the cheaper STC 4304CB, a rebased variant of the more traditional WE 304A of fig. 1D. Meanwhile Western Electric had introduced new double-ended doorknobs which could operate in the middle of a Lecher line system. The 368A was usable as oscillator up to 1.750 MHz. It was also made as 3B/250A by the related British STC. A larger triode was the 388A, capable of 50 W plate dissipation. In 1940 STC proposed variants of the 388A fitted with oxide-coated cathode, to drastically increase emission in pulse applications. But by now England had more advanced solutions available to generate strong pulses at even higher frequencies and from June 1941 the doorknob tubes entered in the black list for new designs.
Fig. 4 - British doorknobs or ‘giant acorns’. A) The STC 4316A was identical to the 316A made by the sister company WE. B) Mullard was related to Philips but used its own coding system. Their TY04-30, identical to the Philips TB04/8, differs from the 316A mainly for its thick pins. C) This double ended prototype, variant of STC 3B/250, evidences an oxide-coated unipotential cathode. Probably proposed in the second half of 1940, after the successful tests of this solution in the Megaw’s cavity magnetron.
Although with more or less significant departures from the original Bell design, doorknob tubes found concrete application in Germany in the Seetakt marine and coastal-watching radar family. First GEMA designed enhanced variants of the WE 316A, the TS1 and the TS1a, the latter one with specular pinout, so that the pair could be mounted back-to-back at both ends of the resonant lines. Plate power dissipation was increased to 40 W and filament current was raised to 6 A in order to increase emission. Seetakt was first installed in 1939 aboard of the Graf Spee. A couple of triodes in the push-pull oscillator delivered pulses of about 1.5 kW at 375 MHz. Such a power proved to be too low, hence from 1941 GEMA introduced the more powerful doorknob triode TS6, with eight parallel wires as cathode and 150 W plate dissipation. A push-pull of TS6 tubes generated 8 kW pulses. This tube was also manufactured in Italy by FIVRE, likely for the ‘EC 3 ter - Gufo’ marine radar made by SAFAR from 1943.
Fig. 5 - German doorknob UHF triodes. A) TS1 and TS1a, with the specular pinout, were improved copies of the WE 316A introduced in 1939. B) A pair of TS6 replaced the couple TS1 and TS1a in the Seetakt transmitter from 1941. Electrodes were deeply redesigned, with eight parallel filaments to increase emission and a finned plate to grant 150 W dissipation. The strengthened structure visible in C) could withstand heavy mechanical shocks, even those caused by the ship's artillery fire.
Fig. 6 - The TU 106 transmitter unit of Seetakt, with two triodes TS6 mounted back-to-back in the push-pull oscillator. The image is reproduced from the US manual TME 11-219, ‘Directory of German Radar Equipment’. According to the today available sources, this unit provided 8 to 15 kW pulses at 375 MHz.
To be continued....
To thank the Author because you find the post helpful or well done.
Part two - Silica valves for low-frequency systems
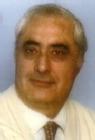
We have seen the early developments of airborne radar sets by the Bowen group. He had to operate at highest possible frequency, mainly to contain the size and hence the drag of the antenna system. As a consequence, he had to accept heavy compromises in the transmitter power. For other applications it was preferable to operate at higher power and then at lower frequencies. Early airborne radars as other types for military needs were designed and built by a joint force team stationed since 1936 at Bawdsey Manor. Due to the secrecy surrounding radio-localization, until 1938 development of suitable transmitting tubes was entirely carried out at the HM Signal School in Portsmouth. It was the same structure where, at the end of the Great War, a group leaded by Captain Stanley R. Mullard, who later founded the homonymous tube factory, started building silica valves. The group was still active as supplier of silica tubes for the communication sets of the Admiralty. No wonder than that the first generation of British transmitters for the new systems was based upon silica valves, readily modifying existing types in order to increase their insulation and emission.
For about twenty years, from 1920 to the second world war, silica valves were the pride of British high-power technology. Until 1918 bulbs of transmitting triodes were made of glass, which softened at relatively low temperature. Even if the bulb was large and spaced from the plate enough to reduce heat transmission, power could not exceeded about 400 W. One of the properties of quartz was the ability to withstand high temperatures, softening above 1.000ºC. Even infrared transmission was very good, an attractive property to facilitate plate heat dissipation by radiation. Mullard had operated before at Edison Swan, developing arc lamps, therefore he decided to use silica bulbs for high-power transmitting tubes. Except for the tungsten filament, which in any case was heated to white heat, all other parts of silica valves were designed to operate at temperatures high enough to melt or soften the corresponding parts of any other vacuum tube. Metal parts were of molybdenum, which melts above 2.600ºC. The basket-like plate structure was made of tiny ribbons double-wound on thick ribs, which were firmly anchored to silica donuts fused to the bulb wall. The discontinuous plate surface was semi-transparent to the radiation from the filament, which only partially contributed to its heating. Even the grid was made of molybdenum, its wire being entwined with three or four molybdenum ribs. Higher power water-cooled silica valves were also made, anode being a spiral wound copper tube. Silica valves were difficult to manufacture and so expensive that defective units had to be returned to shops equipped for repair.
Plate power dissipation could range approximately from 1 to 15 kW. Size was compact, with considerable benefits at high-frequencies. In 1935, when England started designing early radio-localization sets, the choice for silica transmitting tubes was obvious. Since transmitter had to operate in pulsed mode, peak emission of the tungsten filament was the main limiting factor, by far more severe than plate power dissipation. More or less in the same days other countries had decided to develop their own systems at frequencies well beyond the upper limits of power tubes then available. Bench prototypes worked with the WE 316A but for systems to be fully operative, they had to wait until about 1939, when suitable VHF/UHF triodes were released by tube manufacturers. Much time was spent to solve the problems arising during field tests, as the grid emission when heavily driven positive during RF pulses, the sturdiness of electrodes or even simple insulation issues at high plate voltage. On the contrary in England early systems were designed for frequencies that could be handled by available components, with only minor refinements. Systems were readily operative, contributing to the possibility of evaluating their performance and then to program future evolutions based upon the gained experience. As result, in 1939 other nations appeared to be more advanced, with their UHF radar systems better looking than the old-fashioned English ones. But, without the conditioning of other countries for the rush to obtain results, vacuum tube technologies had progressed more than elsewhere and England was ready to release from 1939 onwards a whole series of innovations, which would culminate in microwave systems.
Going back to 1935, the first system evaluation took place using NT41 short-wave transmitting triodes, slightly modified to withstand 5 kV plate voltage. Filament was over-volted to increase emission.
Fig. 7 - Silica valves were the most efficient air-cooled transmitting tubes available in England in the mid 1930s. Designed at the end of the Great War by the HM Signal School in Portsmouth, their bulb was made of fused quartz, capable of withstand temperatures as high as 1.000ºC. Anode was a double-wound basket made of molybdenum ribbon with three or four molybdenum ribs fixed to the bulb. The grid was made of tiny molybdenum wire, while the filamentary cathode was made of tungsten. Their relatively small size contributed to satisfactory operation at high frequencies. Left, with its 250 W plate power dissipation, VT30 was one of the more powerful transmitting triode with glass bulb at the time of early silica valves. Right, typical 2.5 kW silica transmitting tube made for communication equipment. The bulb diameter measures 90 mm.
The first triode specifically intended for pulsed operation in radar transmitters was the NT57, designed in 1936 by H.G. Hughes at the Signal School. Two V-shaped thick tungsten filaments generated 3.6 A minimum emission. Its electrode assembly was considerably short, to operate well above 45 MHz. Long, well spaced and divergent silica stems for plate and grid connections granted effective insulation up to 10 kV. A couple of NT57 was used from 1937 the transmitters of the CH system (Air Ministry Experimental Station or AMES Type 1). Two NT57s were used in the 50 MHz transmitter of MB2, mobile ground system designed in 1937 at Bawdsey for RAF. The same tubes were used in the Type 79 early warning marine radar, operating at 40 MHz, and in the GL1 and GL2 gun laying systems for the Army. Pulse power greater than 20 kW was readily obtainable from a couple of NT57 triodes in the 7.5 m range.
Early in 1939, NT57 evolved in the improved NT57D, with shorter graded-glass seals and emission increased to 5 A. Soon later GEC proposed the NT57T, using thoriated-tungsten filament to raise cathode emission to 18 A, while cutting the filament power from 720 to 315 W. A pair of NT57T triodes generated 70 kW peak pulses in the Type 279 marine radar, successor of the Type 79.
In 1937 Signal School released first samples of a bigger silica valve, the NT60 tetrode which could operate at relatively low anode voltage. Due to its large emitting surface, cathode emission of NT60 was very high, to the point that two tubes in push-pull gave over than 100 kW, about five times greater than that given by a pair of NT57. The NT60 was followed in 1939 by the NT77, with thoriated-tungsten filament and emission increased to 70 A.
Fig. 8 - A) Drawing showing the construction of the NT57 triode, the first silica valve specifically designed for radar applications. Well spaced electrodes and divergent sealing legs grant low capacitance and high insulation. The thick double hairpin filamentary cathode gives 5 A minimum emission under pulsed conditions. B) The photo show a sample of NT57D, with short graded-glass seals, usable up to about 100 MHz. C) It was replaced from April 1939 by the NT57T with thoriated-tungsten filament, capable of delivering 18 A saturated emission, consuming less than half the power required by the filament of the previous one. In D) a sample of CV14 (XN), the latest of compact silica triodes proposed in 1941 to operate up to 240 MHz.
In Summer 1939 Foley at the Signal School designed a large variant of the NT57, bulb size raised to 100 mm diameter by 300 mm length. With its three hairpin-shaped thoriated-tungsten filaments, the NT86 was specified for the hard to believe emission of 90 A at 50 kV anode voltage. The triode could handle 4.5 MW input pulses, probably a never surpassed figure for a radiation-cooled tube. In the Type 281 early warning radar a push-pull of NT86 generated 1 MW pulses, when operated at 60 A, 28 kV input. Another monster silica triode, the NT78, was designed to operate as pulser in the modulator. Its development was difficult, due to overheating of the grid, heavily driven positive during conduction pulses. Input and output power of the Type 281 set was limited by maximum ratings of the modulator, to prevent the onset of phenomena of grid emission and surface evaporation which caused filament poisoning. Type 281 radar remained operational until the end of war. A silica modulator tetrode was also developed, remaining operative until the early sixties, when it was replaced by a much smaller hydrogen thyratron. It was the CV313: three of them in parallel generated the 2 MW pulse to drive the magnetron in the Naval Type 992 radar. Silica triodes intended to operate around 200 MHz, as the XL and XN (CV14) were also experimented, but they never worked properly and any further development was canceled. The reasons for the cancellation of the silica valves were largely due to the enormous production difficulties, which made them incompatible with the increased military demand after the outbreak of the war. Anyway they had performed their task up to 1940 and in some cases even beyond, allowing the many fixed, mobile and naval systems to be fully operative, with pulse power as high as 1 megawatt.
To be continued....
To thank the Author because you find the post helpful or well done.